Ultrastructural immunocytochemistry shows impairment of RNA pathways in skeletal muscle nuclei of old mice: A link to sarcopenia?
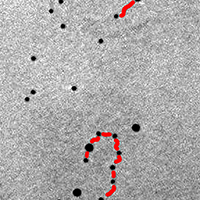
Accepted: 15 March 2021
HTML: 8
All claims expressed in this article are solely those of the authors and do not necessarily represent those of their affiliated organizations, or those of the publisher, the editors and the reviewers. Any product that may be evaluated in this article or claim that may be made by its manufacturer is not guaranteed or endorsed by the publisher.
Authors
During aging, skeletal muscle is affected by sarcopenia, a progressive decline in muscle mass, strength and endurance that leads to loss of function and disability. Cell nucleus dysfunction is a possible factor contributing to sarcopenia because aging-associated alterations in mRNA and rRNA transcription/maturation machinery have been shown in several cell types including muscle cells. In this study, the distribution and density of key molecular factors involved in RNA pathways namely, nuclear actin (a motor protein and regulator of RNA transcription), 5-methyl cytosine (an epigenetic regulator of gene transcription), and ribonuclease A (an RNA degrading enzyme) were compared in different nuclear compartments of late adult and old mice myonuclei by means of ultrastructural immunocytochemistry. In all nuclear compartments, an age-related decrease of nuclear actin suggested altered chromatin structuring and impaired nucleus-to-cytoplasm transport of both mRNA and ribosomal subunits, while a decrease of 5-methyl cytosine and ribonuclease A in the nucleoli of old mice indicated an age-dependent loss of rRNA genes. These findings provide novel experimental evidence that, in the aging skeletal muscle, nuclear RNA pathways undergo impairment, likely hindering protein synthesis and contributing to the onset and progression of sarcopenia.
How to Cite
PAGEPress has chosen to apply the Creative Commons Attribution NonCommercial 4.0 International License (CC BY-NC 4.0) to all manuscripts to be published.