Organotypic spinal cord cultures: An in vitro 3D model to preliminary screen treatments for spinal muscular atrophy
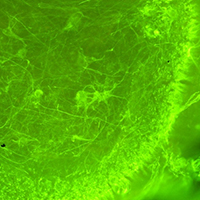
Accepted: 24 August 2021
HTML: 19
All claims expressed in this article are solely those of the authors and do not necessarily represent those of their affiliated organizations, or those of the publisher, the editors and the reviewers. Any product that may be evaluated in this article or claim that may be made by its manufacturer is not guaranteed or endorsed by the publisher.
Authors
Spinal muscular atrophy (SMA) is a severe neuromuscular disease affecting children, due to mutation/deletion of survival motor neuron 1 (SMN1) gene. The lack of functional protein SMN determines motor neuron (MN) degeneration and skeletal muscle atrophy, leading to premature death due to respiratory failure. Nowadays, the Food and Drug Administration approved the administration of three drugs, aiming at increasing the SMN production: although assuring noteworthy results, all these therapies show some non-negligible limitations, making essential the identification of alternative/synergistic therapeutic strategies. To offer a valuable in vitro experimental model for easily performing preliminary screenings of alternative promising treatments, we optimized an organotypic spinal cord culture (derived from murine spinal cord slices), which well recapitulates the pathogenetic features of SMA. Then, to validate the model, we tested the effects of human Mesenchymal Stem Cells (hMSCs) or murine C2C12 cells (a mouse skeletal myoblast cell line) conditioned media: 1/3 of conditioned medium (obtained from either hMSCs or C2C12 cells) was added to the conventional medium of the organotypic culture and maintained for 7 days. Then the slices were fixed and immunoreacted to evaluate the MN survival. In particular we observed that the C2C12 and hMSCs conditioned media positively influenced the MN soma size and the axonal length respectively, without modulating the glial activation. These data suggest that trophic factors released by MSCs or muscular cells can exert beneficial effects, by acting on different targets, and confirm the reliability of the model. Overall, we propose the organotypic spinal cord culture as an excellent tool to preliminarily screen molecules and drugs before moving to in vivo models, in this way partly reducing the use of animals and the costs.
How to Cite

This work is licensed under a Creative Commons Attribution-NonCommercial 4.0 International License.
PAGEPress has chosen to apply the Creative Commons Attribution NonCommercial 4.0 International License (CC BY-NC 4.0) to all manuscripts to be published.