Novel understanding on genetic mechanisms of enteric neuropathies leading to severe gut dysmotility
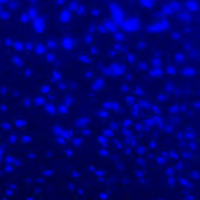
Accepted: 3 November 2021
HTML: 33
All claims expressed in this article are solely those of the authors and do not necessarily represent those of their affiliated organizations, or those of the publisher, the editors and the reviewers. Any product that may be evaluated in this article or claim that may be made by its manufacturer is not guaranteed or endorsed by the publisher.
Authors
The enteric nervous system (ENS) is the third division of the autonomic autonomic nervous system and the largest collection of neurons outside the central nervous system (CNS). The ENS has been referred to as “the brain in the gut” or “the second brain of the human body” because of its highly integrated neural circuits controlling a vast repertoire of gut functions, including absorption/secretion, splanchnic blood vessels, some immunological aspects, intestinal epithelial barrier, and gastrointestinal (GI) motility. The latter function is the result of the ENS fine-tuning over smooth musculature, along with the contribution of other key cells, such as enteric glia (astrocyte like cells supporting and contributing to neuronal activity), interstitial cells of Cajal (the pacemaker cells of the GI tract involved in neuromuscular transmission), and enteroendocrine cells (releasing bioactive substances, which affect gut physiology). Any noxa insult perturbing the ENS complexity may determine a neuropathy with variable degree of neuro-muscular dysfunction. In this review, we aim to cover the most recent update on genetic mechanisms leading to enteric neuropathies ranging from Hirschsprung’s disease (characterized by lack of any enteric neurons in the gut wall) up to more generalized form of dysmotility such as chronic intestinal pseudo-obstruction (CIPO) with a significant reduction of enteric neurons. In this line, we will discuss the role of the RAD21 mutation, which we have demonstrated in a family whose affected members exhibited severe gut dysmotility. Other genes contributing to gut motility abnormalities will also be presented. In conclusion, the knowledge on the molecular mechanisms involved in enteric neuropathy may unveil strategies to better manage patients with neurogenic gut dysmotility and pave the way to targeted therapies.
How to Cite

This work is licensed under a Creative Commons Attribution-NonCommercial 4.0 International License.
PAGEPress has chosen to apply the Creative Commons Attribution NonCommercial 4.0 International License (CC BY-NC 4.0) to all manuscripts to be published.